How little things called Quantum Dots can help tackle Climate Change
Alison Romaine - Writer
Quantum mechanics describes the very smallest things in our universe: molecules, atoms and subatomic particles e.g. electrons, protons and neutrons - the fundamental make-up of our daily lives. It has a ‘strangeness’ (Ghose, 2015) that can make its principles such as ‘superposition’ - where something can be in two places at once - seem so remote from our counterintuitive understanding (based on our ‘real-world’ experience). It is therefore such a departure from the ‘classical’ physics of Newton, that we simply reserve its difficulty for the brainy physicists to handle. However, quantum theory is not just bound to formulae on a blackboard, in fact, it holds up our modern society and proffers solutions to 21st century challenges.
Importance of Quantum Mechanics
Without the understanding of quantum physics, we would not have computers, LEDs (rely on semiconductors) GPS technology (rely on atomic clocks), MRI machines, encryption and lasers which beam through fibre-optic cable to transmit web traffic and handle our Googling! Quantum is also shaping the future - with research in computing (on the verge of quantum ‘supremacy’ or ‘advantage’), drug development and simulation (Marr, 2017). Quantum mechanics can also provide explanation and mitigation to climate change through quantum dot solar cells that are able to absorb otherwise wasted infrared light; increasing power generation (UoT, 2017).
Before the climate change discussion unfolds, a very brief summary of quantum theory and its principles is helpful (as adapted from Walliman, 2019). In quantum physics, objects, let’s say electrons, are described with wave functions in the ‘hidden’ quantum realm, but when we measure them in the world we see, it ‘collapses’ and they become particles. This leads to the ‘particle-wave duality’ principle - the collapse or barrier between them is called the ‘measurement problem’. According to the Copenhagen Interpretation (there are many others) of Bohr and Heisenberg, we don’t have the physics to describe how it does this, hence the ‘problem’! The consequences of these wave functions then introduce the associated quantum principles below:
Superposition
Dirac (1947) described the ‘peculiar relationships’ between states in a system. A wave can be superimposed (added) onto another wave. An electron therefore has the probability of being in different positions on this wave function and thus in ‘two places at once’! You can see this when dropping a pebble in water and seeing waves subsequently overlap. Semiconductors and quantum computing rely on this understanding.
Entanglement
Discussed by Einstein et al., (1935) but defined by Schrödinger (1935), it involves the entanglement of particles such as electrons which can then be described by one wave function. Therefore, when one electron is measured, it instantaneously provides the measurement of its partner; even if it is billions of miles away. This involves ‘non-locality’ (an alien concept to classical physics) as its properties are not local to a physical place but dependent on something remote (Walliman, 2019). It can be used for large scale data mining- with huge implications for health data, smart cities and climate data (Ghose, 2015).
Quantum Tunneling
The phenomenon where electrons, as a wave function, can pass through a barrier that they cannot surpass according to classical physics (Vuille, 2008). Quantum tunnelling enables the sun to shine - while protons typically repel each other, they have a probability to ‘tunnel’ to produce deuterium which turns hydrogen → helium; releasing fusion energy and enabling life one earth (Walliman, 2019).
Heisenberg's Uncertainty Principle (1927)
A wave function contains information on the position (given by amplitude) and momentum (given by wavelength) of an electron. It is a fundamental property of the universe’ (Walliman, 2019) that you either know the position (via a probability distribution) or you know the momentum (if its a sine wave of constant wavelength) of an electron; or you’re in an intermediate state of not knowing both!
Energy Quantization
Quanta is a ‘packet’ of something. Einstein (1905), suggested radiation exists in spatially, discrete packets he called ‘quanta of light’; instead of a continuous flow. Planck also postulated this with electromagnetic energy (Klein, 1961). These quanta-discrete-packet effects were first seen on the atomic spectra where atoms give off light with specific, distinct energies - hence light emitted by an atom is presented like a barcode.
Armed with the latter principle (energy quantization - Figure 1), quantum mechanics can explain the greenhouse gas effect. Meanwhile, its efficient photoelectric application via the tiny ‘quantum dot’ offers a cost-competitive, renewable climate-solution (Calderone, 2018).
Quanta as ‘packet energy’ also occurs in matter (Ghose, 2015). Atoms do not absorb or emit a continuous spectrum of energies. In fact, they instead have very specific energies and thus colours of light that they can absorb (solar cells) or emit (LEDs):
Wavelengths are quantised to certain values - like if you pluck a string with the ends tied together, they can only vibrate in certain restricted ways. This happens to an electron in an atom - the electron wave is quantised to certain wavelengths it can absorb or emit as it is constrained by the atom (adapted from Walliman, 2019).
Quantum physics is able to calculate the energies that respond to these natural rotations and vibrations. When earth re-emits the energy (from the sun) in the form of infrared radiation, quantum mechanics can calculate that it indeed matches with the quantised energy of CO2 molecules and other greenhouse gases - hence heat absorption by an electron; which then escapes. Conveniently, the matching of quantised energy can be applied and augmented for photoelectric effects in solar cells.
These tiny, semiconductor nanocrystals (Figure 3)(silicon is comparatively bulkier) yield three electrons from the absorption of one high-energy photon of sunlight; today’s photovoltaic solar cells yield, at most, one electron from a photon; the remaining energy is lost as heat (Calderone, 2018). Quantum Dot Solar Cells (QDSC) can therefore be three times more efficient than existing solar cells which achieve only 33% conversion of the sun’s energy → electricity (Calderone, 2018) (Figure 4). P-type (+ charged holes) and N-type (excess of electrons) junctions are the building blocks of all solar cells and LEDs. Rabani in Patel (2011) states how doping (adding small quantities of other elements) enables a p-n junction creation which separates electrons and holes that otherwise quickly combine in QDs when a photon is absorbed.

Figure 3: Quantum Dot Structure
Source Image: University of Toronto
Quantum mechanics describes the very smallest things in our universe: molecules, atoms and subatomic particles e.g. electrons, protons and neutrons - the fundamental make-up of our daily lives. It has a ‘strangeness’ (Ghose, 2015) that can make its principles such as ‘superposition’ - where something can be in two places at once - seem so remote from our counterintuitive understanding (based on our ‘real-world’ experience). It is therefore such a departure from the ‘classical’ physics of Newton, that we simply reserve its difficulty for the brainy physicists to handle. However, quantum theory is not just bound to formulae on a blackboard, in fact, it holds up our modern society and proffers solutions to 21st century challenges.
Importance of Quantum Mechanics
Without the understanding of quantum physics, we would not have computers, LEDs (rely on semiconductors) GPS technology (rely on atomic clocks), MRI machines, encryption and lasers which beam through fibre-optic cable to transmit web traffic and handle our Googling! Quantum is also shaping the future - with research in computing (on the verge of quantum ‘supremacy’ or ‘advantage’), drug development and simulation (Marr, 2017). Quantum mechanics can also provide explanation and mitigation to climate change through quantum dot solar cells that are able to absorb otherwise wasted infrared light; increasing power generation (UoT, 2017).
Before the climate change discussion unfolds, a very brief summary of quantum theory and its principles is helpful (as adapted from Walliman, 2019). In quantum physics, objects, let’s say electrons, are described with wave functions in the ‘hidden’ quantum realm, but when we measure them in the world we see, it ‘collapses’ and they become particles. This leads to the ‘particle-wave duality’ principle - the collapse or barrier between them is called the ‘measurement problem’. According to the Copenhagen Interpretation (there are many others) of Bohr and Heisenberg, we don’t have the physics to describe how it does this, hence the ‘problem’! The consequences of these wave functions then introduce the associated quantum principles below:
Superposition
Dirac (1947) described the ‘peculiar relationships’ between states in a system. A wave can be superimposed (added) onto another wave. An electron therefore has the probability of being in different positions on this wave function and thus in ‘two places at once’! You can see this when dropping a pebble in water and seeing waves subsequently overlap. Semiconductors and quantum computing rely on this understanding.
Entanglement
Discussed by Einstein et al., (1935) but defined by Schrödinger (1935), it involves the entanglement of particles such as electrons which can then be described by one wave function. Therefore, when one electron is measured, it instantaneously provides the measurement of its partner; even if it is billions of miles away. This involves ‘non-locality’ (an alien concept to classical physics) as its properties are not local to a physical place but dependent on something remote (Walliman, 2019). It can be used for large scale data mining- with huge implications for health data, smart cities and climate data (Ghose, 2015).
Quantum Tunneling
The phenomenon where electrons, as a wave function, can pass through a barrier that they cannot surpass according to classical physics (Vuille, 2008). Quantum tunnelling enables the sun to shine - while protons typically repel each other, they have a probability to ‘tunnel’ to produce deuterium which turns hydrogen → helium; releasing fusion energy and enabling life one earth (Walliman, 2019).
Heisenberg's Uncertainty Principle (1927)
A wave function contains information on the position (given by amplitude) and momentum (given by wavelength) of an electron. It is a fundamental property of the universe’ (Walliman, 2019) that you either know the position (via a probability distribution) or you know the momentum (if its a sine wave of constant wavelength) of an electron; or you’re in an intermediate state of not knowing both!
Energy Quantization
Quanta is a ‘packet’ of something. Einstein (1905), suggested radiation exists in spatially, discrete packets he called ‘quanta of light’; instead of a continuous flow. Planck also postulated this with electromagnetic energy (Klein, 1961). These quanta-discrete-packet effects were first seen on the atomic spectra where atoms give off light with specific, distinct energies - hence light emitted by an atom is presented like a barcode.
Armed with the latter principle (energy quantization - Figure 1), quantum mechanics can explain the greenhouse gas effect. Meanwhile, its efficient photoelectric application via the tiny ‘quantum dot’ offers a cost-competitive, renewable climate-solution (Calderone, 2018).
Quanta as ‘packet energy’ also occurs in matter (Ghose, 2015). Atoms do not absorb or emit a continuous spectrum of energies. In fact, they instead have very specific energies and thus colours of light that they can absorb (solar cells) or emit (LEDs):
Wavelengths are quantised to certain values - like if you pluck a string with the ends tied together, they can only vibrate in certain restricted ways. This happens to an electron in an atom - the electron wave is quantised to certain wavelengths it can absorb or emit as it is constrained by the atom (adapted from Walliman, 2019).
Quantum physics is able to calculate the energies that respond to these natural rotations and vibrations. When earth re-emits the energy (from the sun) in the form of infrared radiation, quantum mechanics can calculate that it indeed matches with the quantised energy of CO2 molecules and other greenhouse gases - hence heat absorption by an electron; which then escapes. Conveniently, the matching of quantised energy can be applied and augmented for photoelectric effects in solar cells.
Quantum mechanics enables customisation of energy levels by engineering what (light) artificial atoms (you can not change the quantised energy levels of existing atoms) can absorb and emit. Atoms can be designed to maximise the amount of solar energy that is converted to electricity - increasing cell efficiency (Ghose, 2015). Currently, standard silicon cells are unable to tap into the infrared region which constitutes half of our incoming sunlight. If we tap into just 1% of the sun’s ‘yottawatts’ it produces, we would suffice the planetary energy needs (Ghose, 2015). Efficiency reduces costs and makes renewable-energy alternatives increasingly viable and it’s all down to…
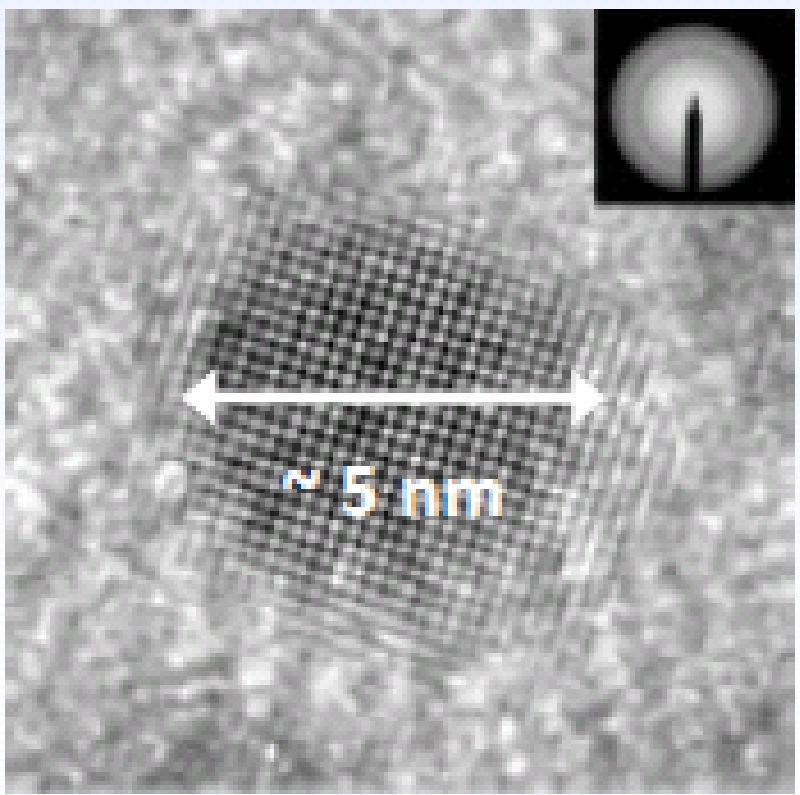
Figure 2: TEM of PbSe quantum dot. Quantum Dots can create printable thin-film solar cells.
Source: Jasim in IntechOpen (2014)
… the (5nm) Quantum Dot (Figure 2). The new type of solar cell.
Figure 2: TEM of PbSe quantum dot. Quantum Dots can create printable thin-film solar cells.
Source: Jasim in IntechOpen (2014)
… the (5nm) Quantum Dot (Figure 2). The new type of solar cell.
How do Quantum Dots differ from other captivating photovoltaic materials?
These tiny, semiconductor nanocrystals (Figure 3)(silicon is comparatively bulkier) yield three electrons from the absorption of one high-energy photon of sunlight; today’s photovoltaic solar cells yield, at most, one electron from a photon; the remaining energy is lost as heat (Calderone, 2018). Quantum Dot Solar Cells (QDSC) can therefore be three times more efficient than existing solar cells which achieve only 33% conversion of the sun’s energy → electricity (Calderone, 2018) (Figure 4). P-type (+ charged holes) and N-type (excess of electrons) junctions are the building blocks of all solar cells and LEDs. Rabani in Patel (2011) states how doping (adding small quantities of other elements) enables a p-n junction creation which separates electrons and holes that otherwise quickly combine in QDs when a photon is absorbed.
Figure 3: Quantum Dot Structure
Source Image: University of Toronto
How do Quantum Dots work?
Quantum dots are artificially made atoms (Ashoori, 1996) that have a bandgap (the minimum energy required to excite an electron to a state where it can conduct - PV Education, 2015 ) which is defined by adjusting its size or shape (AltEnergyMag, 2018). The bandgap can be tuned from the visible → to the infrared- matching the solar spectrum (Patel, 2011); all from a single material system. Lead sulfide colloidal quantum dots (Figure 4) enable infrared energy to be as equally accessible (NREL, 2017).

Figure 4: Structure of a Quantum Dot Solar Cell
Source: AltEnergyMag (2018)
Quantum dots acquire surplus photon energy that is otherwise lost as heat. Light rays enter through the transparent electrode (Figure 5a) onto a light absorbing layer of dots to generate electron hole pairs. The charged particles then separate (Figure 5b) and travel to respective electrodes; inducing an electric current.

Figure 5a: Schematic Workings of a (Schottky barrier) Quantum dot solar cell
Figure 5b: Band diagram of Schottky solar cell:
Source: Jasim in IntechOpen (2014)
Figure 4: Structure of a Quantum Dot Solar Cell
Source: AltEnergyMag (2018)
Quantum dots acquire surplus photon energy that is otherwise lost as heat. Light rays enter through the transparent electrode (Figure 5a) onto a light absorbing layer of dots to generate electron hole pairs. The charged particles then separate (Figure 5b) and travel to respective electrodes; inducing an electric current.
Figure 5a: Schematic Workings of a (Schottky barrier) Quantum dot solar cell
Figure 5b: Band diagram of Schottky solar cell:
Source: Jasim in IntechOpen (2014)
Quantum dot solar cells have a collection of benefits (Figure 6).
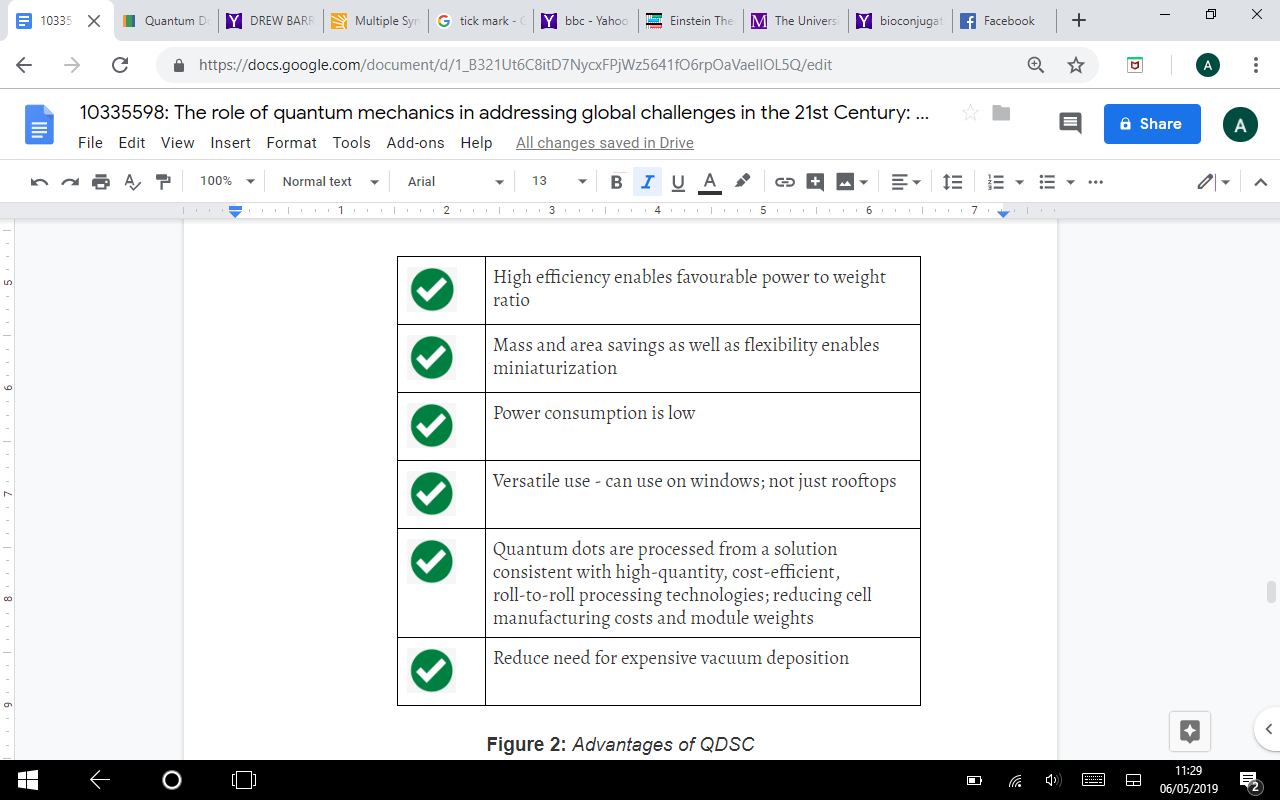
Figure 6: Advantages of QDSC
Source: Information adapted from AltEnergyMag, 2018
In correspondence with increased renewable electricity generation, we can apply the same quantum mechanic principle in order to minimise our consumption of energy (20% of energy we use is for lighting - Ghose, 2015) by customising what LEDs (light emitting diodes) can emit. We can now build emitters that produce white light more efficiently; operating at energy levels 5% of regular bulbs (Ghose, 2015).
Hence, there is huge potential to have cleaner energy generation through exploiting solar power and lower energy consumption through super efficient LEDs. However, before QDs are released into the commercial market, there are some hiccups. Cadmium and selenium ions, used in the QD core, are known to be cytotoxic (harmful to living cells). However, bioconjugation - the coupling of biomolecules by a covalent bond - increases the size of these nanometre dots (Calderone, 2018); preventing cell permeation.
While the brain-bending workings of quantum physics operate at a scale where unfamiliar phenomena happen (even Einstein was uncomfortable with entanglement as it has a flavour of faster-than-light communication… which is not possible in his theory of relativity), it is the fundamental make-up of our everyday lives. Companies are investing in quantum technology - for example Toronto based QD Solar announced its intention to develop QD solar material that can be applied to any flexible surface to generate energy (2017) - so watch out! We should attempt to rethink quantum mechanics not as an arcane, exclusive science (it’s perhaps clear that I am no physicist!) but appreciate its application as it is deployed to tackle the challenges of the 21st century.
Sources:
Ashoori, R. C. (1996). "Electrons in artificial atoms". Nature. 379 (6564): 413–419.
Calderone, L. (2018). Quantum Dot Solar Cells Are Coming | AltEnergyMag. [online] Altenergymag.com. Available at: https://www.altenergymag.com/article/2018/05/quantum-dot-solar-cells-are-coming/28547 [Accessed Apr. 2019].
Dirac P.A.M. (1947). The Principles of Quantum Mechanics (2nd edition). Clarendon Press. p. 12.
Einstein A, Podolsky B, Rosen N; Podolsky; Rosen (1935). "Can Quantum-Mechanical Description of Physical Reality Be Considered Complete?" (PDF).
Einstein, A. (1905). "Über einen die Erzeugung und Verwandlung des Lichtes betreffenden heuristischen Gesichtspunkt" 17 (6): 132–148.
Ghose, S. (2015). How Quantum Physics Can Help Us Fight Climate Change | Shohini Ghose | TEDxVictoria. [online] YouTube. Available at: https://www.youtube.com/watch?v=x02ZKWkexeo [Accessed Apr. 2019].
Jasim (2014). Quantum Dot Solar Cells. [online] IntechOpen. Available at: https://www.intechopen.com/books/solar-cells-new-approaches-and-reviews/quantum-dots-solar-cells [Accessed Apr. 2019].
Klein, Martin J. (1961). "Max Planck and the beginnings of the quantum theory". Archive for History of Exact Sciences. 1 (5): 459
Kurzgesagt (2018) "Quantum Computers Explained – Limits of Human Technology". youtube.com.. 8 December 2017. Accessed April 2019.
Marr, B. (2017). 6 Practical Examples Of How Quantum Computing Will Change Our World. [online] Forbes.com. Available at: https://www.forbes.com/sites/bernardmarr/2017/07/10/6-practical-examples-of-how-quantum-computing-will-change-our-world/ [Accessed Apr. 2019].
Patel, P. (2011). Quantum Dots as Solar Cells. [online] MIT Technology Review. Available at: https://www.technologyreview.com/s/423528/quantum-dots-as-solar-cells/ [Accessed Apr. 2019].
PV Education. (2015). Band Gap [Online]. Available: http://www.pveducation.org/pvcdrom/pn-junction/band-gap
Schrödinger E (1935). "Discussion of probability relations between separated systems". Mathematical Proceedings of the Cambridge Philosophical Society. 31 (4): 555–563.
University of Toronto News. (2017). U of T startup QD Solar secures international financing. [online] Available at: https://www.utoronto.ca/news/u-t-startup-qd-solar-secures-international-financing [Accessed Apr. 2019].
Vuille (2008). College Physics. 2 (Eighth ed.). Belmont: Brooks/Cole.
Walliman, D. (2019). Quantum Physics, Try This!. [online] YouTube. Domain of Science. Available at: https://www.youtube.com/watch?v=Usu9xZfabPM&t=667s [Accessed Apr. 2019].
Walliman, D. (2019). The Interpretations of Quantum Mechanics. [online] YouTube. Available at: https://www.youtube.com/watch?v=mqofuYCz9gs [Accessed Apr. 2019].
Zeilinger A (1999). "Experiment and the foundations of quantum physics". Rev. Mod. Phys. 71 (2): S288–S297.
Figure 6: Advantages of QDSC
Source: Information adapted from AltEnergyMag, 2018
In correspondence with increased renewable electricity generation, we can apply the same quantum mechanic principle in order to minimise our consumption of energy (20% of energy we use is for lighting - Ghose, 2015) by customising what LEDs (light emitting diodes) can emit. We can now build emitters that produce white light more efficiently; operating at energy levels 5% of regular bulbs (Ghose, 2015).
Hence, there is huge potential to have cleaner energy generation through exploiting solar power and lower energy consumption through super efficient LEDs. However, before QDs are released into the commercial market, there are some hiccups. Cadmium and selenium ions, used in the QD core, are known to be cytotoxic (harmful to living cells). However, bioconjugation - the coupling of biomolecules by a covalent bond - increases the size of these nanometre dots (Calderone, 2018); preventing cell permeation.
While the brain-bending workings of quantum physics operate at a scale where unfamiliar phenomena happen (even Einstein was uncomfortable with entanglement as it has a flavour of faster-than-light communication… which is not possible in his theory of relativity), it is the fundamental make-up of our everyday lives. Companies are investing in quantum technology - for example Toronto based QD Solar announced its intention to develop QD solar material that can be applied to any flexible surface to generate energy (2017) - so watch out! We should attempt to rethink quantum mechanics not as an arcane, exclusive science (it’s perhaps clear that I am no physicist!) but appreciate its application as it is deployed to tackle the challenges of the 21st century.
Sources:
Ashoori, R. C. (1996). "Electrons in artificial atoms". Nature. 379 (6564): 413–419.
Calderone, L. (2018). Quantum Dot Solar Cells Are Coming | AltEnergyMag. [online] Altenergymag.com. Available at: https://www.altenergymag.com/article/2018/05/quantum-dot-solar-cells-are-coming/28547 [Accessed Apr. 2019].
Dirac P.A.M. (1947). The Principles of Quantum Mechanics (2nd edition). Clarendon Press. p. 12.
Einstein A, Podolsky B, Rosen N; Podolsky; Rosen (1935). "Can Quantum-Mechanical Description of Physical Reality Be Considered Complete?" (PDF).
Einstein, A. (1905). "Über einen die Erzeugung und Verwandlung des Lichtes betreffenden heuristischen Gesichtspunkt" 17 (6): 132–148.
Ghose, S. (2015). How Quantum Physics Can Help Us Fight Climate Change | Shohini Ghose | TEDxVictoria. [online] YouTube. Available at: https://www.youtube.com/watch?v=x02ZKWkexeo [Accessed Apr. 2019].
Jasim (2014). Quantum Dot Solar Cells. [online] IntechOpen. Available at: https://www.intechopen.com/books/solar-cells-new-approaches-and-reviews/quantum-dots-solar-cells [Accessed Apr. 2019].
Klein, Martin J. (1961). "Max Planck and the beginnings of the quantum theory". Archive for History of Exact Sciences. 1 (5): 459
Kurzgesagt (2018) "Quantum Computers Explained – Limits of Human Technology". youtube.com.. 8 December 2017. Accessed April 2019.
Marr, B. (2017). 6 Practical Examples Of How Quantum Computing Will Change Our World. [online] Forbes.com. Available at: https://www.forbes.com/sites/bernardmarr/2017/07/10/6-practical-examples-of-how-quantum-computing-will-change-our-world/ [Accessed Apr. 2019].
Patel, P. (2011). Quantum Dots as Solar Cells. [online] MIT Technology Review. Available at: https://www.technologyreview.com/s/423528/quantum-dots-as-solar-cells/ [Accessed Apr. 2019].
PV Education. (2015). Band Gap [Online]. Available: http://www.pveducation.org/pvcdrom/pn-junction/band-gap
Schrödinger E (1935). "Discussion of probability relations between separated systems". Mathematical Proceedings of the Cambridge Philosophical Society. 31 (4): 555–563.
University of Toronto News. (2017). U of T startup QD Solar secures international financing. [online] Available at: https://www.utoronto.ca/news/u-t-startup-qd-solar-secures-international-financing [Accessed Apr. 2019].
Vuille (2008). College Physics. 2 (Eighth ed.). Belmont: Brooks/Cole.
Walliman, D. (2019). Quantum Physics, Try This!. [online] YouTube. Domain of Science. Available at: https://www.youtube.com/watch?v=Usu9xZfabPM&t=667s [Accessed Apr. 2019].
Walliman, D. (2019). The Interpretations of Quantum Mechanics. [online] YouTube. Available at: https://www.youtube.com/watch?v=mqofuYCz9gs [Accessed Apr. 2019].
Zeilinger A (1999). "Experiment and the foundations of quantum physics". Rev. Mod. Phys. 71 (2): S288–S297.
Comments
Post a Comment